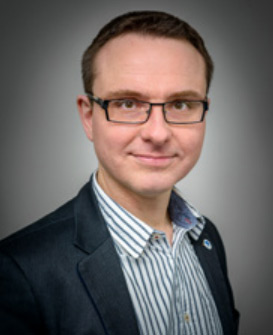 |
Janusz M. Bujnicki, PhD, Professor
Correspondence address: Laboratory of Bioinformatics and Protein Engineering International Institute of Molecular and Cell Biology 4 Ks. Trojdena Street, 02-109 Warsaw, Poland Email: This email address is being protected from spambots. You need JavaScript enabled to view it. www: iimcb.genesilico.pl tel: +48 (22) 597 0750; fax: +48 (22) 597 0715
|
DEGREES
2009 - Professor of Biological Sciences, nomination by the President of the Republic of Poland
2005 - DSc Habil in Biochemistry, Institute of Biochemistry and Biophysics, Polish Academy of Sciences, Warsaw, Poland
2001- PhD in Biology, University of Warsaw, Faculty of Biology, Poland
1998 - MSc in Microbiology, University of Warsaw, Faculty of Biology, Poland
PROFESSIONAL EXPERIENCE
2002-present - Professor, Head of Laboratory of Bioinformatics and Protein Engineering, International Institute of Molecular and Cell Biology in Warsaw, Poland (100% appointment)
2019-present - Scientific Advisor, Łukasiewicz Research Network - PORT Polish Center for Technology Development (25% appointment)
2006-2020 - Associate Professor (extraordinarius), Bioinformatics Laboratory, Institute of Molecular Biology and Biotechnology, Adam Mickiewicz University, Poznań, Poland
2010-2011 - Deputy Director, International Institute of Molecular and Cell Biology in Warsaw (1 year rolling position)
2008 - Visiting Professor, University of Tokyo, Japan(sabbatical)
2004-2006 - Assistant Professor, Adam Mickiewicz University, Poznań, Poland
2001 - Visiting Scientist, National Center for Biotechnology Information,National Institutes of Health, Bethesda, Maryland, USA
1999-2002 - Research Scientist, Bioinformatics Laboratory, International Institute of Molecular and Cell Biology in Warsaw, Poland
1998-2000 - Senior Research Assistant, Henry Ford Hospital, Detroit, Michigan, USA
SELECTED PROFESSIONAL AFFILIATIONS
2019-2022 - Member, Committee for Science Evaluation, Ministry of Science and Higher Education
2020 - Member, Advisory Group on Preventing, Counteracting and Combating COVID-19, Ministry of Science and Higher Education
2019-present - Member, University Council, University of Warsaw (Chairman, 2019-2020)
2018-present - Member, Academia Europaea
2018-present - Member, European Molecular Biology Organization
2017-present - Member, European Science Advisors Forum
2016-present - Corresponding Member, Polish Academy of Sciences
2016-2017 - Member, Council of the National Science Congress
2015-2020 - Member, Group of Chief Scientific Advisors, European Commission’s Scientific Advice Mechanism
2014-2018 - Member, Scientific Policy Committee, Polish Ministry of Science and Higher Education
2013-present - Executive Editor, Nucleic Acids Research
2013-2016 - Member, Scientific Committee of the Innovative Medicines Initiative
2013-2015 - Member, Science Europe: Life, Environmental and Geo Sciences (LEGS) Scientific Committee
2011-2016 - Member, Polish Young Academy, Polish Academy of Sciences
2007-present - Member, Polish Bioinformatics Society (founding member; Vice-President, 2007-2010; President, 2011-2013)
2007-present - Member, RNA Society
2001-present - Member, International Society for Computational Biology (Senior Member, 2015-)
SELECTED AWARDS AND FELLOWSHIPS
2019 - André Mischke Young Academy of Europe Prize for Science and Policy Honorary Award "For Merits for Inventiveness," Prime Minister at the request of the Polish Patent Office
2019 - Award for Organizational Achievements, Ministry of Science and Higher Education
2017 - Crystal Brussels Sprout Award
2015 - Jan Karol Parnas Award of the Polish Biochemical Society
2014 - National Science Centre Award for outstanding scientific achievements
2014 -Master Award, Foundation for Polish Science
2014 - Prime Minister’s Award for outstanding scientific achievements
2014 - Selected as one of “25 leaders for the next 25 years” by Teraz Polska magazine of the Polish Promotional Emblem Foundation
2014 - Knight's Cross of the Order of Polonia Restituta
2014 - Award in the Science category of the national plebiscite “Poles with Verve”
2013 - ERC Proof of Concept Grant
2012 - Award for Outstanding Research Achievements, Ministry of Science and Higher Education
2010 - ERC Starting Grant (2011-2015)
2009 - Scholarship for Outstanding Young Scientists, Minister of Science and Higher Education
2009 - Award for Research Achievements, Ministry of Science and Higher Education
2006 - Prime Minister Award for habilitation thesis
2006 - Young Researcher Award in Structural and Evolutionary Biology, Visegrad Group Academies of Sciences
2003, 2004 - START Scholarship for Young Scientists, Foundation for Polish Science
2002-2005 - EMBO/HHMI Young Investigator Award
2002 - Award for best Polish genetics-related publication in 2002, Polish Genetics Society
2001 - Award for best Polish publication on nucleic acid biochemistry in 2000, Polish Biochemical Society and Sigma-Aldrich
DOCTORATES DEFENDED UNDER LAB LEADER’S SUPERVISION
A. Żylicz-Stachula, A. Chmiel, I. Cymerman, A. Czerwoniec, M. Gajda, M. Pawłowski, J. Sasin-Kurowska, J. Kosiński, A. Obarska-Kosińska, S. Pawlak, E. Purta, K. Tkaczuk, Ł. Kościński, M.Rother, W. Potrzebowski, I. Korneta, T. Puton, J. Kasprzak, I. Tuszyńska, Ł. Kozłowski, M. Werner, A. Kamaszewska, A. Philips, K. Milanowska, M. Piętal, D. Matelska, K. Majorek, M. Domagalski, T. Osiński, M. Machnicka, M. Magnus, K. Szczepaniak, M. Zielińska, Astha, I. Foik, D. Toczydłowska-Socha.
Our group is involved in theoretical and experimental research on sequence structure-function relationships in proteins, nucleic acids, and macromolecular complexes. Theoretical research involves the development of computer software for the analysis of biological macromolecules. We are currently focusing on developing software for the structural prediction and modeling of RNA and RNA protein complexes. To date, we have developed and made publicly available one of the first methods for the automated comparative (template-based) modeling of three-dimensional (3D) RNA structures (ModeRNA; http://iimcb. genesilico.pl/moderna/) and a method for de novo (template-free) RNA structure modeling (SimRNA; http://genesilico.pl/software/stand-alone/simrna, also available as a web server at http://genesilico. pl/SimRNAweb). We also developed a method for predicting metal ion binding sites in RNA structures (MetalionRNA; http://metalionrna.genesilico.pl), a method for modeling RNA-ligand complexes, and a method for predicting the structure of RNA-protein complexes (http://genesilico.pl/NPDock). Other methods for RNA bioinformatics include a method for the classification of contacts in RNA 3D structures (ClaRNA; http://iimcb.genesilico.pl/clarna/) and a method for the flexible superposition of RNA 3D structures and their fragments (SupeRNAlign; http:// genesilico.pl/supernalign/). We also developed various databases, including a database of RNA modification pathways and enzymes (MODOMICS; http://modomics. genesilico.pl) and a database of RNA 3D motifs and their interactions (RNA Bricks; http://iimcb.genesilico.pl/rnabricks/).
Our suite of programs for the prediction and analysis of protein structures and macromolecular complexes includes the GeneSilico MetaServer (https://www.genesilico.pl/meta2/), methods for modeling large macromolecular complexes with the use of restraints that are derived from experimental data (PyRy3D, http://genesilico.pl/pyry3d/; MinkoFit3D, http://iimcb.genesilico.pl/minkofit3d/), and a method for discriminating models according to their agreement with experimental data (FILTREST3D; http://filtrest3d.genesilico.pl/). We also developed methods for predicting order/ disorder in protein structures (http://iimcb. genesilico.pl/metadisorder/).
Our experimental research focuses on elucidating sequence structure-function relationships in bio-macromolecules (currently mainly RNA and RNA-protein complexes, also with small chemical molecules) using biophysics, biochemistry, molecular biology, and cell biology. We tightly integrate theoretical and experimental research. We often experimentally test functional and structural predictions for RNAs, proteins, and their complexes that are obtained using computational methods. For structural studies, we combine X-ray crystallography and low-resolution methods, such as small-angle X-ray scattering (SAXS), structure probing by chemical modification or crosslinking, mass spectrometry, circular dichroism, mutagenesis, etc. Most recently, our group began using cryo-EM. We have collected our first datasets and began developing software for interpreting the data.
RECENT HIGHLIGHTS
Matching tRNA modifications in humans to their known and predicted enzymes he acquisition of post-transcriptional chemical modifications is an essential part of the maturation process, required to generate functional tRNA molecules. Modifications play different roles in controlling the stability, folding, and decoding properties of tRNAs and can be determinants or antideterminants of other components of the translation apparatus, such as aminoacyl-tRNA synthetases. Additionally, tRNA modifications can be recognition elements of ribonucleases, leading to the generation of tRNA fragments that affect multiple cellular processes. However, very few modifications (e.g., m1G37, Ψ55, and t6A37) are present at a specific position of a particular tRNA in (almost) all known organisms. Most of them are specific to particular taxons, from species to kingdoms. For example, lysidine (k2C34) is a hallmark of bacteria, whereas archaeosine (G+15) is only found in archaea. Depending on the organism, the total number of genes that encode tRNA modification enzymes varies from 11 in some obligate symbionts to ~100 in humans, of which 50 are currently represented in MODOMICS, the main database of RNA modification pathways that was developed and is maintained by the Bujnicki group at IIMCB, in collaboration with various groups worldwide (http://modomics.genesilico.pl).
The near complete sets of tRNA modification genes are currently available for only one organism per domain of life: Saccharomyces cerevisiae for eukarya (where only one gene that is required for the formation of ncm5U out of cm5U is missing), Escherichia coli for bacteria (where only the genes for ho5U34 and Acp3U47 formation remain unidentified), and Haloferax volcanii for archaea (where a handful of genes are missing). Beyond these three organisms, the annotation of tRNA modification genes remains scarce because of difficulties in connecting various RNA modification enzymes, which often exhibit complex evolutionary relationships, with various modifications that are present in tRNAs. It has been difficult to identify enzymes that are responsible for many tRNA modifications and hence to determine the function of those tRNA modifications in many species, including humans.
Recently, an increasing number of mutations that cause genetic diseases have been mapped to human genes that encode tRNA modification enzymes, thus making a comprehensive list of these genes highly desirable. In a collaborative effort with Valerie de Crécy-Lagard and her team at the University of Florida, Sebastian Leidel and his team at the University of Bern (previously MPI Muenster and University of Muenster), and Todd Lowe at the University of California, Santa Cruz, we compiled a comprehensive list of known and predicted tRNA modifications in Homo sapiens with genes that are implicated in their biosynthesis. This analysis allowed the identification of remaining gaps in knowledge in the field of human tRNA modifications and will help guide future experiments. Furthermore, we have used publicly available datasets to determine the expression profiles and proteomic evidence of known and predicted modification enzymes. Our work will facilitate access to the current knowledge on human tRNA modification enzymes for a wider community of biologists. These results were published in Nucleic Acids Research (de Crécy-Lagard et al., 2019).
Ongoing work: new bioinformatics methods for predicting structures of RNA-protein complexes
RNA-protein (RNP) interactions play pivotal roles in various biological processes, such as protein synthesis, the regulation of gene expression, RNA splicing, transport, storage, and stabilization. To understand the functional and mechanistic details of these processes, it is essential to have information about the 3D structures of these complexes. The inherent flexibility of RNA molecules and transient nature of these complexes make it technically difficult to determine these structures experimentally. In addition to experimental work, our laboratory develops methods for theoretical predictions.
One of our recent developments is SimRNP, a variant of SimRNA that enables the modeling of interactions between RNA and other types of molecules, in this case proteins. The representation of RNA and statistical potential for RNA are essentially the same, whereas the representation of protein molecules is very similar to the coarse-grained model and statistical potential that are used in the REFINER program for protein folding (developed earlier by Michał Boniecki). Additionally, a statistical potential for RNA-protein interactions was introduced based on an analysis of high-resolution structures of RNAprotein complexes. A typical task for SimRNP is to predict the 3D structure of an RNP complex, starting from an unbound structure of the protein and RNA components or from an unbound protein structure and unfolded RNA sequence. Protein components are typically partially restrained to maintain the protein fold, but conformational changes are allowed to reflect induced fit upon RNA-protein binding.
If the structures of both components (RNA and protein) are available, then the RNP complex structure can be predicted by docking, in which conformational sampling can be separated from scoring of the conformations that are obtained. The existing docking methods can be broadly classified as rigid docking algorithms that do not account explicitly for conformational changes and flexible docking algorithms that attempt to account for conformational changes. Major challenges in RNP docking are molecular flexibility and computational complexity that are associated with flexible docking. Generating conformations that are similar to the bound conformation from the starting structures and discriminating them from others is a challenging task. We developed a prototypical meta-predictor for RNA-protein docking, which combines various existing methods for docking and scoring to obtain biologically, chemically, and physically relevant predictions. Such meta-predictions were successfully applied previously to model protein structures and protein-protein docking. RNP docking is performed using different methods, and the top-scoring docking poses from each of these are rescored using different scoring functions. If the scoring methods reach a consensus, then decoys that are obtained from different methods are clustered together. However, in the absence of consensus scoring, top models that are proposed by different methods are suggested as alternative solutions. The MetaRNPDock metaserver is available at http://genesilico.pl/metaRNPDock/ (co-authors: Nithin Chandran, Sunandan Mukherjee, Pietro Boccaletto, Michał J. Boniecki, and Janusz M. Bujnicki).